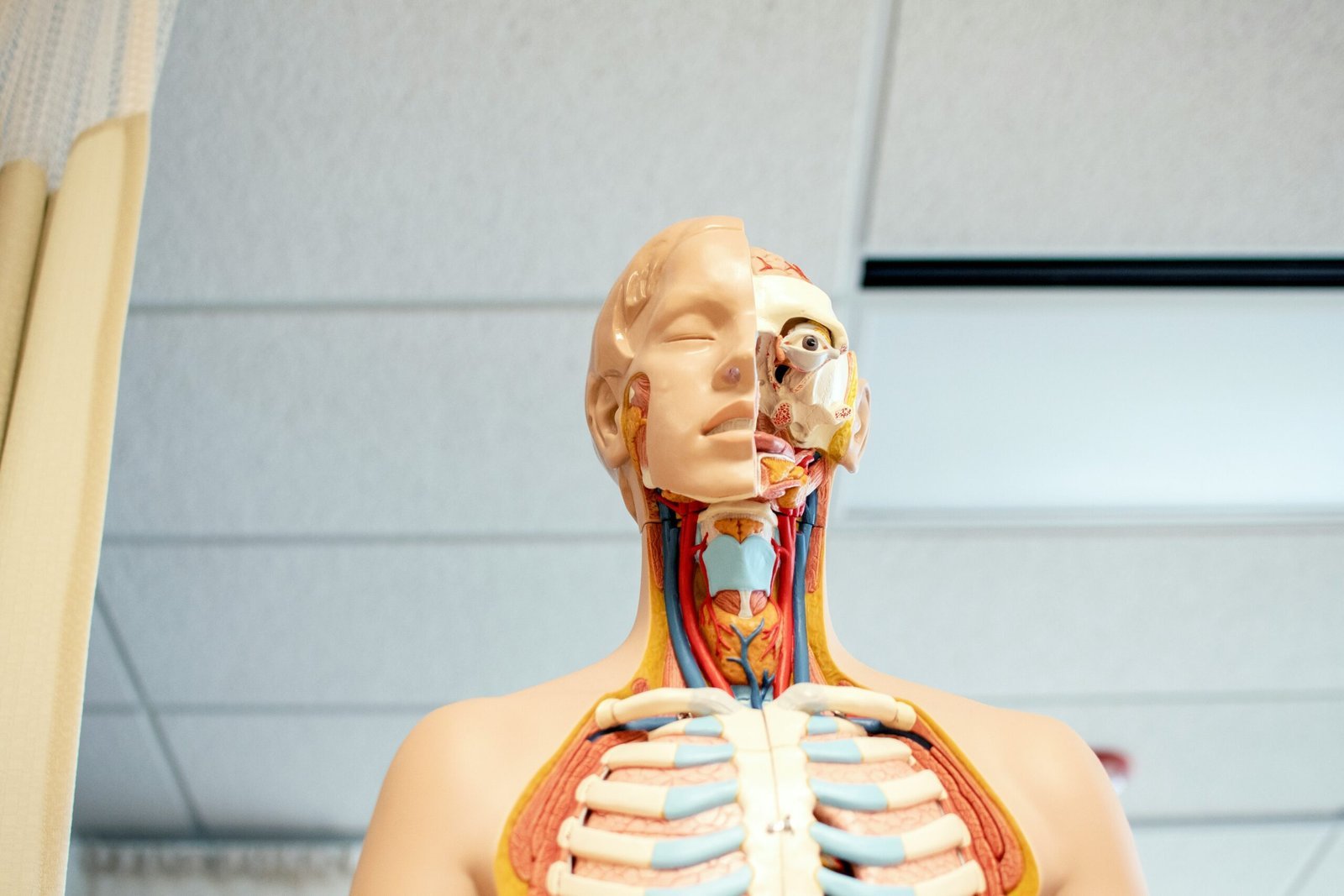
Introduction to Synthetic Biology
Synthetic biology, an emerging and highly interdisciplinary field, represents the confluence of biology, engineering, genetics, and computational science. It involves the design and construction of new biological parts, devices, and systems, as well as the re-design of existing natural biological systems for novel, useful purposes. The ultimate goal of synthetic biology is to understand and harness the principles underlying biological life to create systems that can perform specific, human-defined tasks.
The roots of synthetic biology can be traced back to key historical developments in molecular biology. In the 1970s, the advent of recombinant DNA technology marked a revolutionary milestone, enabling scientists to manipulate genetic material with unprecedented precision. This was further bolstered by the development of polymerase chain reaction (PCR) in the 1980s, which allowed for the amplification of DNA sequences, and the mapping of the human genome in the early 2000s. These breakthroughs laid the groundwork for the birth of synthetic biology as a distinct discipline.
By leveraging tools and methodologies from engineering, synthetic biologists aim to standardize biological parts—akin to electronic components in engineering—thus facilitating the creation of intricate biological circuits and pathways. This standardization is integral to the field, as it attempts to modularize biological components to enable predictable, scalable, and reproducible outcomes. Moreover, advances in computational science have enhanced our ability to model and simulate biological systems, thereby accelerating the design cycle and improving the precision of synthetic biology interventions.
Ultimately, the aspirations driving synthetic biology extend beyond mere scientific curiosity. The potential applications are vast and varied, ranging from developing new medical therapies and diagnostic tools to synthesizing biofuels and creating sustainable agricultural practices. By blending the principles of natural and artificial systems, synthetic biology aims no less than to redefine the boundaries of what is biologically possible.
Technologies and Techniques in Synthetic Biology
Synthetic biology is characterized by an array of advanced technologies and techniques designed to create new biological systems with unprecedented precision and functionality. Central to this endeavor is gene synthesis, which allows for the de novo construction of DNA sequences. Gene synthesis facilitates the creation of customized gene sequences that can be used to generate new proteins, pathways, or entire organisms.
Another cornerstone technology is CRISPR-Cas9 genome editing. This powerful and versatile method enables the precise modification of DNA sequences within a living organism. CRISPR-Cas9 uses a guide RNA to target specific DNA loci, where the Cas9 enzyme introduces cuts. These cuts are subsequently repaired, allowing for precise insertions, deletions, or substitutions in the genome. This technology revolutionizes synthetic biology by enabling the creation of organisms with new or enhanced functionalities.
Complementing these genetic tools are synthetic genomes, which involve designing and constructing entire genomes from scratch. By synthesizing large DNA segments and assembling them into complete genomes, researchers can create organisms with tailored genetic codes. This ambitious approach has the potential to pave the way for custom-designed microbes or plants that could solve complex problems in medicine, agriculture, and industry.
In these sophisticated endeavors, computer-aided design (CAD) tools and software play a crucial role. These tools facilitate the precise design of new biological components by modeling and simulating genetic constructs before they are physically synthesized. CAD tools are essential for tasks such as optimizing gene sequences, predicting protein structures, and ensuring the compatibility of genetic modules.
Fundamental to synthetic biology are modular genetic parts like promoters, enhancers, and terminators. These elements serve as the building blocks for assembling synthetic genetic circuits. For instance, promoters control the initiation of gene transcription, enhancers boost gene expression, and terminators signal the end of transcription. By combining these parts, scientists can construct genetic circuits that perform specific tasks, such as biosensing, metabolic engineering, and programmable cell behavior.
Collectively, these technologies and techniques are crucial in advancing synthetic biology, enabling the creation of innovative and functional biological systems tailored to address various scientific and practical challenges.
Applications of Synthetic Biology
Synthetic biology has emerged as a transformative field with extensive applications across various industries. By engineering and redesigning biological systems, researchers and industry professionals are opening new avenues for innovation and problem-solving.
In the medical field, synthetic biology plays a pivotal role in advancing gene therapy and personalized medicine. Scientists are designing targeted treatments by modifying genes at the cellular level to treat diseases more effectively. For instance, CAR-T cell therapy, which engineers a patient’s own immune cells to combat cancer, is a prime example of how synthetic biology can produce groundbreaking therapeutics. Another remarkable development is the creation of synthetic probiotics tailored to treat gastrointestinal disorders by harboring engineered bacteria that produce therapeutic compounds directly within the gut.
Agriculture is another sector reaping the benefits of synthetic biology. Custom-designed microorganisms are being developed to enhance crop yields and resist pests. Genetically engineered plants are not only more resilient to changing environmental conditions but also reduce the need for harmful pesticides. In addition, synthetic biology facilitates the production of bio-fertilizers that improve soil health organically and sustainably.
Environmental management has also seen significant advancements through synthetic biology, particularly in bioremediation. Engineered microorganisms are adept at breaking down pollutants like oil spills and heavy metals, thus restoring ecosystems more efficiently than traditional methods. These microbes can be customized to target specific contaminants, making them a powerful tool in conservation efforts.
In the realm of manufacturing, synthetic biology is revolutionizing bio-based production processes. Engineered organisms are employed to produce biofuels, decreasing reliance on fossil fuels, and reducing carbon emissions. Moreover, synthetic biology enables the production of complex chemicals and materials, such as bioplastics and pharmaceuticals, in a more sustainable and cost-effective manner compared to traditional industrial methods.
The potential for future applications of synthetic biology is vast. Ongoing research aims to expand its capabilities, exploring areas like synthetic ecosystems, organ regeneration, and even programmable biosensors. As the field continues to evolve, synthetic biology holds promise for addressing some of the world’s most pressing challenges, from healthcare to environmental sustainability.
Ethical, Legal, and Social Implications
Synthetic biology’s transformative potential brings a complex array of Ethical, Legal, and Social Implications (ELSI) that require rigorous examination. One significant concern is biosafety, revolving around the creation and potential release of synthetic organisms. These engineered entities could pose unknown risks to natural ecosystems and human health. The unpredictability of genetic modifications raises the alarm for unanticipated consequences, necessitating robust biosafety protocols to mitigate potential hazards.
Biosecurity is another critical aspect, highlighting the potential misuse of synthetic biology. The prospect of creating novel pathogens or modifications that could be weaponized underscores the need for comprehensive biosecurity measures. Policymakers, scientists, and law enforcement agencies must collaborate to establish stringent monitoring and control mechanisms to prevent malicious applications. Continuous vigilance is essential to safeguard against biosecurity threats posed by advancements in synthetic biology.
Diverse stakeholder perspectives must be considered when assessing ethical implications. Scientists are at the frontier, tasked with navigating the fine line between groundbreaking innovation and ethical responsibility. Policymakers are charged with creating practical yet stringent regulatory frameworks to oversee the development and application of synthetic biology technologies. The public’s role is equally vital, as societal acceptance hinges on transparent dialogue and informed consent. Public engagement ensures the societal values and concerns are reflected in policy formation.
Ethical considerations extend to the moral status of synthetic life forms. The possibility of creating synthetic entities with life-like properties elicits questions about their moral and legal standing. Determining the rights and ethical treatment of such organisms demands careful contemplation. Additionally, the potential for unintended consequences from synthetic biology innovations necessitates precautionary measures to prevent adverse outcomes.
Existing regulatory frameworks encompass varied standards across regions, highlighting the need for coherent global policies. Initiatives such as the Cartagena Protocol on Biosafety provide a foundation, yet need adaptation to the nuances of synthetic biology. Proposed policies should emphasize the responsible development, ensuring scientific benefits do not overshadow ethical obligations and societal welfare.