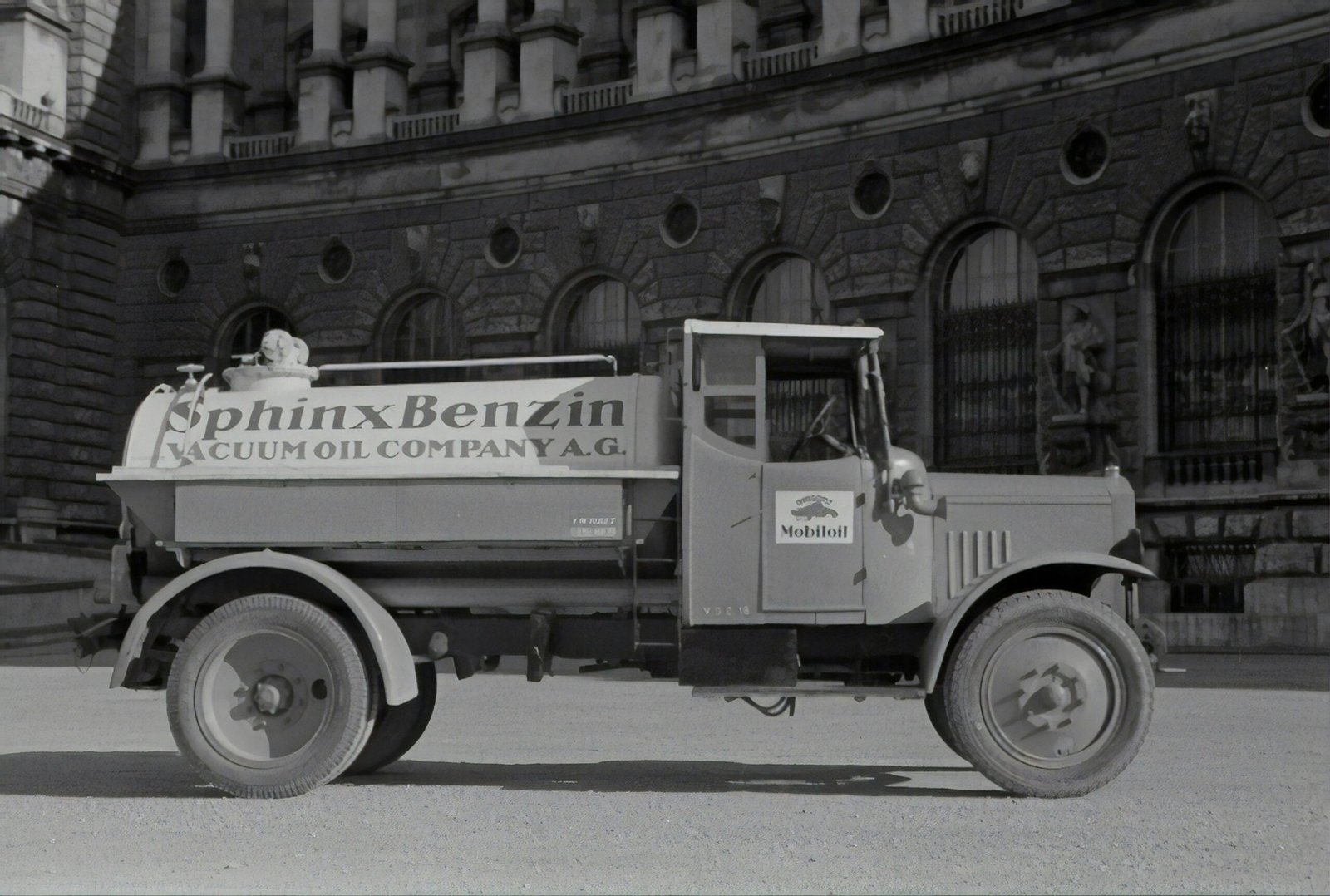
Introduction to Bioenergy
Bioenergy represents a pivotal component in the landscape of renewable energy resources. Defined broadly, bioenergy is energy derived from organic matter, or biomass, including plant and animal materials, agricultural residues, and even certain types of waste. Its significance extends beyond merely providing an alternative energy source; bioenergy plays a crucial role in reducing our dependency on fossil fuels, thus offering a pathway to a more sustainable and eco-friendly energy future.
Historically, humanity has utilized biomass in its most rudimentary form—burning wood and other plant materials for warmth and cooking. This practice dates back millennia and underscores the fundamental role that organic matter has played in human survival and development. However, the advent of industrialization and the subsequent rise in energy demands prompted a shift towards more efficient and technologically advanced methods of harnessing bioenergy.
In the 20th and 21st centuries, technological advancements have revolutionized bioenergy production, moving from simple combustion methods to sophisticated processes such as anaerobic digestion, gasification, and the creation of liquid biofuels like ethanol and biodiesel. These modern bioenergy technologies offer enhanced efficiency and have significantly lowered the greenhouse gas emissions associated with energy production. Importantly, bioenergy can be integrated into existing energy infrastructures, making it a versatile option for reducing carbon footprints.
The role of bioenergy in mitigating climate change cannot be overstated. By displacing fossil fuel usage, bioenergy contributes to lower levels of carbon dioxide and other harmful pollutants in the atmosphere. Furthermore, as a renewable resource, bioenergy can be continuously replenished through sustainable agricultural and forestry practices. This cyclical nature of bioenergy production ensures a balanced carbon cycle, where the carbon released during energy production is offset by the carbon absorbed during biomass growth.
As we confront the escalating challenges of climate change and dwindling fossil fuel reserves, the importance of incorporating bioenergy into our comprehensive renewable energy strategy becomes ever more apparent. Its historical evolution and modern advancements underscore bioenergy’s potential to serve as a cornerstone of sustainable energy systems moving forward.
How Bioenergy is Produced
Bioenergy production involves converting organic matter, known as feedstocks, into usable forms of fuel through a variety of methods. This process can yield different types of biofuels, including ethanol, biodiesel, and biogas. Each type of biofuel requires specific production processes and feedstocks, emphasizing both sustainability and efficiency.
One common method of producing bioenergy is anaerobic digestion. This process breaks down organic matter in the absence of oxygen, producing biogas, a mixture of methane and carbon dioxide. Anaerobic digestion is particularly effective for materials like agricultural residues and animal manure. The methane produced can be used for heating, electricity generation, or as a vehicle fuel, contributing to a circular economy by recycling waste products.
Fermentation is another key process, primarily used to produce ethanol. In this method, microorganisms such as yeast convert carbohydrates found in plant materials like sugarcane, corn, and cellulosic biomass into alcohol. Ethanol can then be blended with gasoline to create a biofuel that reduces greenhouse gas emissions when compared to conventional fossil fuels. The fermentation process is not only efficient but also scalable, making it a vital component of the global bioenergy landscape.
Biodiesel production involves a chemical process called transesterification, where fats and oils are reacted with alcohol to produce fatty acid methyl esters (FAME), commonly known as biodiesel. Feedstocks for biodiesel are diverse, ranging from vegetable oils to animal fats and even recycled cooking grease. This versatility in feedstock choice enhances the sustainability aspect of biodiesel, as it allows for the utilization of waste materials that would otherwise be disposed of.
The types of feedstocks used in bioenergy production are crucial to its sustainability. Agricultural residues, forestry waste, and dedicated energy crops all play significant roles. Agricultural residues such as straw and corn stover can be converted into biofuels without interfering with food production, while forestry waste offers a sustainable use for materials left over from logging activities. Energy crops like switchgrass and miscanthus are specifically grown for their high biomass yield, further enhancing the efficiency of biofuel production.
By leveraging these various methods and feedstocks, bioenergy production not only provides a renewable source of energy but also contributes to waste reduction and environmental sustainability. The integration of bioenergy into the broader energy mix is essential for achieving a more sustainable and resilient energy future.
Bioenergy, derived from organic matter, serves as a versatile and sustainable alternative to fossil fuels, finding applications across various sectors including transportation, electricity generation, and heating. In the transportation sector, biofuels such as biodiesel and bioethanol are being increasingly adopted to power vehicles, reducing reliance on traditional petroleum-based fuels. The integration of advanced biofuels, such as cellulosic ethanol and algae-based fuels, is pivotal in achieving stringent renewable energy targets and minimizing carbon footprints.
Electricity generation is another critical application of bioenergy. Biomass power plants convert organic materials like agricultural residues, wood chips, and animal waste into electricity through processes such as combustion, gasification, and anaerobic digestion. This not only provides a steady supply of renewable energy but also helps in managing waste, turning potential environmental pollutants into valuable resources.
In the realm of heating, bioenergy is employed in various forms, including biogas used in combined heat and power (CHP) systems, and biomass pellets consumed in residential and industrial boilers. Such applications contribute to energy diversification and reduce dependency on coal and natural gas, fostering a more resilient and sustainable energy system.
The benefits of bioenergy extend beyond environmental sustainability. By mitigating greenhouse gas emissions, bioenergy plays a crucial role in addressing climate change. Moreover, bioenergy enhances energy security by diversifying energy sources and reducing the vulnerability associated with fossil fuel imports. The utilization of locally available biomass resources supports rural economic development, creating jobs in agriculture, forestry, and bioenergy production sectors.
Furthermore, the development and deployment of advanced biofuels are driving innovation within the energy industry. These cutting-edge technologies not only improve the efficiency and sustainability of bioenergy production but also open new avenues for integrating bioenergy with other renewable energy sources, such as solar and wind, creating a more cohesive and sustainable energy landscape.
Challenges and Future Prospects of Bioenergy
The bioenergy sector faces significant hurdles that impact its sustainability and efficiency. One of the primary challenges is the consistent supply of feedstock. Ensuring a steady and economical supply of biomass materials is pivotal but complicated by seasonal variations, logistical obstacles, and varying quality of raw materials. Furthermore, the industry grapples with the intricate issue of land use. The allocation of agricultural land for energy crops often competes directly with land needed for food production, leading to a critical food vs. fuel debate.
Economic challenges also play a crucial role. High initial investment costs for bioenergy technologies, along with fluctuating market prices for both biofuels and fossil fuels, hinder large-scale adoption. Moreover, technological advancements are necessary to enhance the efficiency and cost-effectiveness of bioenergy production. Innovations in conversion technologies, like advanced enzymatic processes, and improvements in the energy density of biofuels could potentially mitigate some of these economic pressures.
Despite these challenges, the future prospects for bioenergy remain promising. Ongoing research and development endeavors are critical to unlocking new potentials. For instance, advancements in genetic engineering could improve biomass yield and resistance to environmental stresses, while cutting-edge biotechnologies could lead to more efficient conversion processes. Additionally, the development of integrated biorefineries that produce multiple bioproducts alongside biofuels could enhance overall economic viability.
Policy frameworks will play a pivotal role in shaping the future of bioenergy. Government incentives, subsidies, and carbon pricing mechanisms can create a more conducive environment for growth. International cooperation and standardization can further harmonize efforts, fostering a more globally cohesive bioenergy sector.
In essence, while the bioenergy industry faces notable challenges, continued innovation and supportive policy measures offer a promising pathway toward a sustainable and scalable renewable energy source.